Millions of years in the making: How our eyes see a fastball
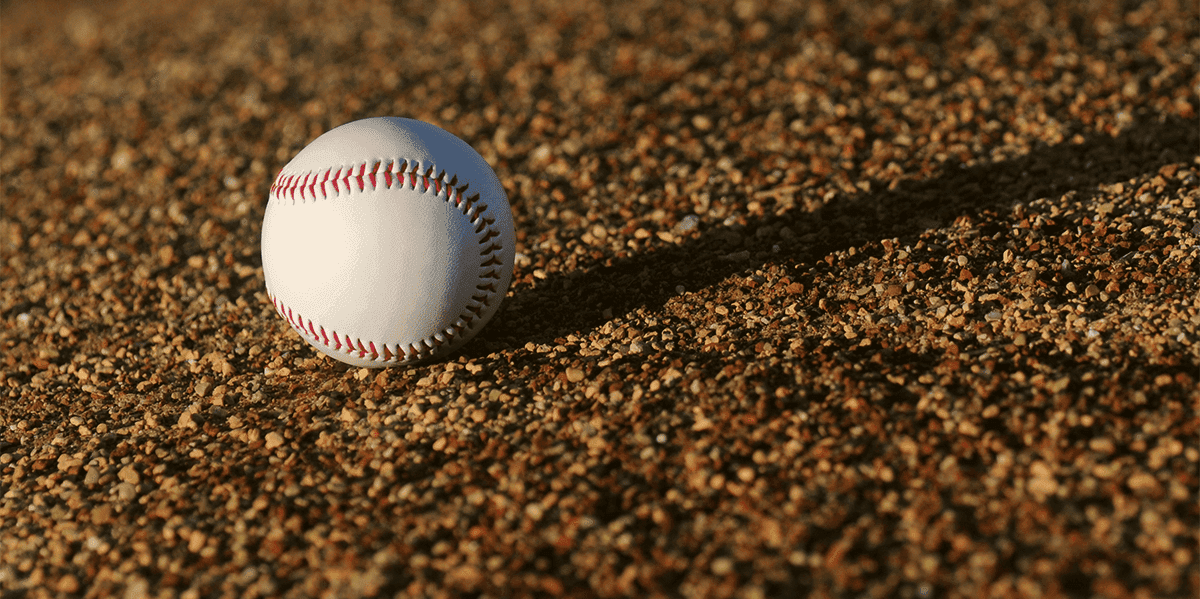
Baseball fan or not, late October can be an electrifying time. Some of the world’s best players go head-to-head in a game that challenges the limits of the human body. As the pitcher launches a fastball down the mound, how fast can the batter’s neurons react? How well can the batter’s eyes track the ball? It’s in these moments where millions of years of evolution are really put to the test.
The dirt beneath her cleats crunches faintly as she walks onto the field. She’s nervous, but confident. Years of practice have given her the ability to track a fast-moving baseball, whether it’s rocketing off the end of a bat or the hand of a pitcher. The practice that gave Josalyn these abilities didn’t start when she was a kid. Far from it. It started millions of years ago, before humans even existed.
It’s hard to say exactly when lifeforms first gained the ability to see, but estimates suggest it may have been as long as 1.5 billion years ago. It’s believed that some early, bacteria-like lifeforms had a segment of DNA that told them how to build a light-sensitive protein—a rhodopsin-like molecule. Rhodopsins are a type of protein that help specialized cells in our eyes sense different colors of light. It’s not clear exactly why this primitive version initially developed, but one possibility is that light helped them navigate to places where there was enough sunlight to give them energy without harming them1,2.
Whatever the actual benefit was, the ability to sense light was clearly helpful and it was here to stay. Over hundreds of millions of years, primitive eyes continued to evolve, eventually giving rise to an incredible spectrum of different eye types1,2. Some eyes evolved to help nocturnal animals see at night, while others evolved the ability to detect various colors.
Your DNA may impact your body’s response to exercise. These products can help you learn about it.
“Batter up!” The umpire’s booming voice is her cue. She steps into the batter’s box and looks up to the pitcher standing 60 feet away. That’s a good distance, but she knows the average fastball can cross it in about 0.4 seconds. Not to worry: Josalyn’s eyes are more than capable of seeing whatever the pitcher can throw.
Unlike the first eyes to see light, hers are made of millions of specialized cells, each of which have a special role to play in vision. Humans are unique among primates with our large eyes showing large amount of white tissue3. Scientists believe this may have evolved as a way to socialize and communicate with facial expression, but the white portion of the eye—the sclera—has a more basic function, too. The sclera helps give our eye structure and shape, which enables it to focus light where it needs to be4.
It takes 0.2 seconds to see the ball and swing
Set in contrast to the sclera, the central parts of the eye are alive with color. The iris contains a number of specialized cells, some of which produce a brown pigment known as melanin. Eyes with large amounts of melanin will appear to be a shade of brown. Those with little melanin may seem blue. The blue is a bit of an illusion—there’s no blue pigment, it’s just that without melanin to absorb the light, it penetrates to a slightly deeper part of the iris where the blue waves of light are scattered3. Why we have pigmented irises is a bit of a mystery, but some evidence suggests that it helps shield our vision from excess light. Light that can penetrate through the eye runs the risk of producing a glare that obscures our vision, so the iris may help prevent this3.
At the center of the eye is the pupil. Its dark color is the result of light-absorbing proteins in the back of the eye. The size of the pupil—and thus the amount of light entering the eye—is determined by muscles in the iris that contract to make the pupil smaller, or relax to make it bigger4. Light enters the pupil and is focused directly onto the fovea, part of the retina, at the back of the eye. In this area, there are multiple layers of neurons that produce light-sensing proteins—similar to those made by the earliest lifeforms. Opsins and melanopsin proteins sense the light and trigger electric pulses that travel from neuron to neuron. Eventually these electric waves reach the brain, where they’re interpreted as an image2,4.
It takes time for all of this to happen. Research suggests that it’ll take Josalyn’s brain about 0.2 seconds to register the pitch, and respond with a swing5.
Her eyes follow the ball as it’s released from the pitcher’s hand, laces spinning just so. It’s in this moment where the game of baseball challenges her and the millions of years of genetic practice behind her by asking one question: Are you advanced enough to react? Tiny muscles in her iris had already relaxed, increasing the size of the pupil to bring in more light on a dark night. The brown in her iris gives her extra protection from the glare of the stadium lights. Everything and everyone is focused on the speeding ball. Her brain registers it, anticipates its trajectory, and sets a swing into motion.
Want to learn how your DNA may affect traits like your eye color? Learn more here!
- “Light and the evolution of vision” Eye (London, England) vol. 30,2 (2015): 173-8.
- Arendt, Detlev et al. “The ‘division of labour’ model of eye evolution” Philosophical transactions of the Royal Society of London. Series B, Biological sciences vol. 364,1531 (2009): 2809-17.
- Sturm, Richard A., and Mats Larsson. “Genetics of Human Iris Colour and Patterns.” Pigment Cell & Melanoma Research, vol. 22, no. 5, 2009, pp. 544–562., doi:10.1111/j.1755-148x.2009.00606.x.
- Kolb, Helga. “Gross Anatomy of the Eye.” Webvision: The Organization of the Retina and Visual System [Internet]., U.S. National Library of Medicine, 1 May 2007, www.ncbi.nlm.nih.gov/books/NBK11534/.
- Higuchi, Takatoshi et al. “Contribution of Visual Information about Ball Trajectory to Baseball Hitting Accuracy” PloS one vol. 11,2 e0148498. 5 Feb. 2016, doi:10.1371/journal.pone.0148498